I know, it sounds like an absurd question. Most people think of life in terms of, you know, things with arms or legs or tentacles. Most of the experts will tell you that water is the most important prerequisite for life. They’re wrong.
Way back in 1944, the eminent physicist Erwin Schroedinger published a book entitled “What is Life?” in which he laid out the fundamental principles governing the existence of life. I’m going to review and expand upon his ideas, which to this day remain little understood. That’s because his definition of life is based on thermodynamics, and we all know that thermodynamics is impenetrable.
Entropy and Negentropy
The central notion here is that of entropy and its opposite, negentropy. Roughly speaking, entropy is disorderliness and negentropy is orderliness. Here are some pictures that illustrate the concepts.
27 51 88 97 15 25 87 38 47 17 61 22 76 69 2 4 6 8 10 12 14 16 18 20 22 24 26 28 30 32
;ow irhj -qpojlkam a,pcai dlaa ,a,v. aa.w a million monkeys with a million typewriters
If you haven’t gotten a feeling for what entropy and negentropy are by this point, you might as well give up and stop reading this essay; go read a comic book.
Let’s get a little more formal now. Suppose that you were to put 10 pennies into a box, shake the box, and dump the pennies onto the table. What are the odds that you’d get all heads? Pretty low, right? And all heads would correspond to lots of negentropy, right? What are the odds that you’d get 5 heads and 5 tails, or 6 of one and 4 of the other? A lot higher, right? And that arrangement would correspond to a high entropy situation. Thus, we have a quick and dirty way of estimating how much negentropy something has: how improbable that something would be if we randomly shuffled its parts.
Let’s apply that principle. Suppose that we put Chris into a wood chipper:
We collect the red goo that comes out; we now have 150 pounds of red liquid. Suppose that we were to shake up that red goo and dump it out. What are the odds that we’d end up with Chris? Obviously, the odds are extremely low that we’d end up with Chris. This means that Chris is something with lots and lots of negentropy.
Negentropy and Life
We can apply the same test to any living creature, and reach the same result: every living creature is an extremely unlikely arrangement of its atoms. All living systems have loads of negentropy. This gives us our first solid characterization of life: it has lots of negentropy. Not water — negentropy.
Of course, my computer has lots of negentropy, too. If we put it through a wood chipper, then tried to put it back together again, the odds of doing so are also extremely low. So negentropy by itself does not suffice to define life.
We can now add another observation: Chris, unlike his computer, is always falling apart. Every day his jowls hang a little lower, his wrinkles grow a little larger, and his mind loses some more of its cogency. Indeed, if Chris did nothing to resist this trend, in just a few days, he’d be dead. But Chris has the means to counter this trend, to maintain his high level of negentropy:
Yes, friends, Chris drinks chocolate milk every day. Of course, chocolate milk isn’t the only way to stave off the loss of negentropy. Food of almost any kind does the job. I just happen to like chocolate milk.
Now, you’ve heard all those ninnies telling you that certain foods give you vitamins, minerals, and energy. That’s true, but of only secondary importance. What’s most important about food is that it gives you negentropy. The energy in food is merely the carrier of the negentropy. The vitamins and minerals are of tertiary importance. Of course, if you want to live a long, happy, and disease-free life, then you’ll need all those vitamins and minerals, too, but the top priority is the negentropy. That’s the most important thing keeping you alive.
So now we have a second fundamental characteristic of living systems: they have the means to collect negentropy from the environment to maintain their highly improbably structure. Notice again that this has nothing to do with water or temperature or any of the other things that people like to cite.
We often hear life defined in terms of the ability to reproduce. Sure, that’s important — but only if you’re going to die. Why should any living system die? Why couldn’t a living system last forever, if it could continue harvesting negentropy? Life here on earth does die, and that’s because death permits better adapted descendants to take the place of the old, obsolete ancestors. But that’s a secondary consideration; fundamentally, there’s no reason why a living system must die, hence no fundamental requirement for reproduction.
So here’s my thermodynamic definition of life, which is really just a restatement of Schroedinger’s definition:
A system is alive if it has high negentropy and the capability to harvest enough negentropy to maintain itself for an indefinite period of time.
You can readily see that this definition fits all life on earth. It also fits my speculation about Life in the Sun. And it also, possibly, fits Jupiter’s Great Red Spot.
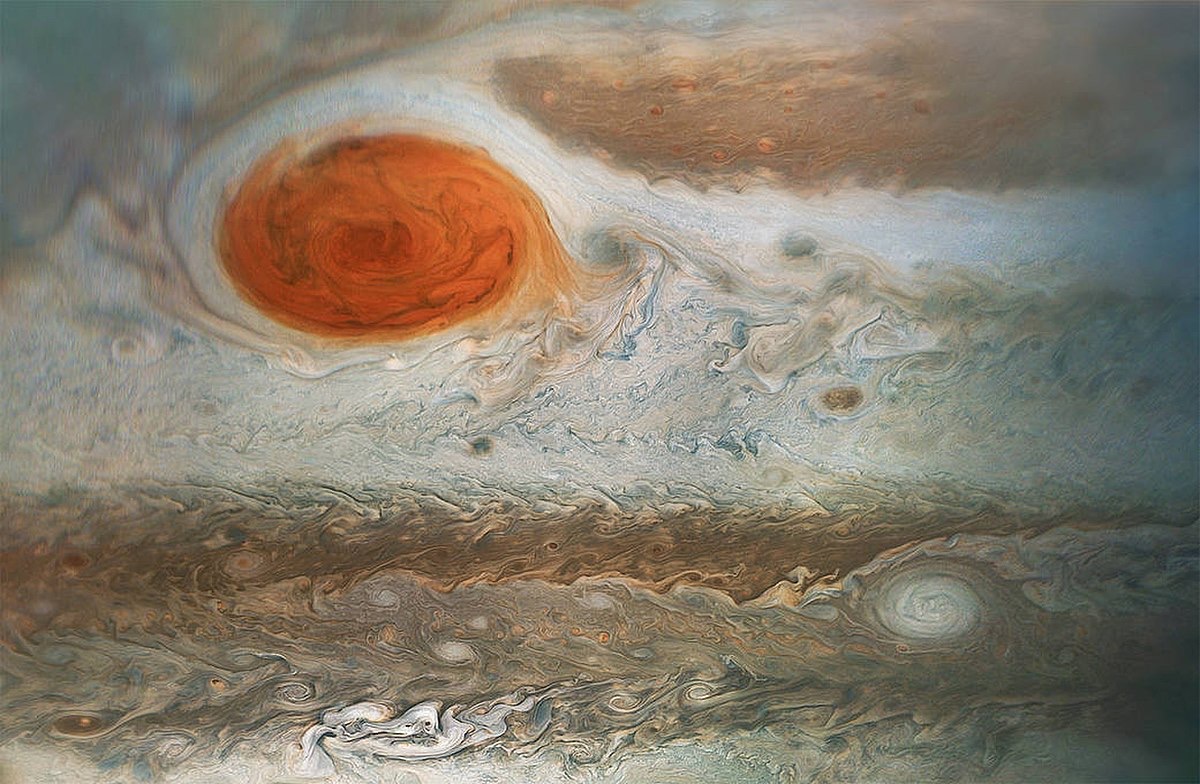
We know that the Great Red Spot is rather like a hurricane: its winds constantly circle around the core. But a hurricane on earth is not alive because it quickly runs out of the negentropy it needs to survive. In fact, it actually destroys negentropy by forming clouds that block the sun that heats the surface of the ocean, and warm water is the source of negentropy for the hurricane. Hence, terrestrial hurricanes are one-shot, self-destroying phenomena.
But the Great Red Spot has been hanging around for a long time; it is at least 200 years old and may be 350 years old. There can be no question that this is a complex system and that it has successfully harvested negentropy during that time. But where does its negentropy come from?
It could be the same negentropy that the earth gets from sunlight, but since Jupiter is so much further away from the sun than the earth, this seems unlikely. A more plausible possibility is the energy being generated internally by Jupiter, about 10**17 watts, several thousand times greater than the energy released by the earth’s interior. This energy is most likely generated by slow compression of the planet, although spotty deuterium fusion in the core might contribute a little energy. If some mechanism were to funnel an excess of this energy in an organized manner, the Great Red Spot might be the result. Thus, the Great Red Spot could well be “alive” in the same sense that a bacterium is alive. Could it be only one manifestation of a larger phenomenon? After all, the complex motions of Jupiter’s belts are also far from thermodynamic equilibrium. Could the Jovian atmosphere as a whole be alive? The thermodynamics suggests that it is possible. We might be able to detect improbable patterns in the various emissions from the Jovian atmosphere.
This is all highly speculative, and until we know more about the internal structure of the planet, it will be impossible to know the causes of the Great Red Spot. But we should not forget that the GRS is unique in the solar system, and that it violates the spherical symmetry that we would expect in a planet like Jupiter, and that we see in Saturn, Uranus, and Neptune.
What are the best and worst places to look for living systems?
Once you make the transition from thinking about life in terrestrial terms and instead think in thermodynamic terms, a whole host of possibilities arise. The earth provides a good starting point. It has a transparent atmosphere that allows the entry of lots of sunlight, and it rotates quickly and has a large mass of ocean water, both of which serve to smooth out temperature fluctuations, maximizing the net input of negentropy. Where else in the universe might we find such conditions, or even better conditions?
The ideal would be a situation with maximum negentropy per unit area. That would be just outside the atmosphere of a star. However, that would maximize the energy per unit area. In order to get the maximum negentropy per unit area, we need a strong temperature difference. Just sitting in front of a star would only produce an extremely high temperature. The trick is to maintain a low temperature close to the star. This can best be accomplished with a rapidly rotating sphere. Anything on the surface would be exposed to intense light, which would begin to heat it up, but before the temperature rose by much, that part of the surface would have rotated into the night side, where it would radiate energy into space and cool down. I won’t drag you through the mathematics for such a situation; it involves some calculus. But this is the same mechanism that permits life on earth. If the earth didn’t rotate, the side facing the sun would be horridly hot and the side facing away from the sun would be very cold. Mercury’s side facing the sun reaches a temperature of 750ºF while the back side is at -330ºF. The difference in these two temperatures represents the immense amount of negentropy available at that distance from the sun — if only those two places could be brought into thermal contact with each other. They can’t.
By contrast, the absolute worst place in the universe to get negentropy would be at the surface of a star’s photosphere. Here we’ve got tremendous amounts of energy leaving at the same temperature as the surface. There’s no temperature difference to grab hold of for negentropy. It’s about as perfectly randomized a physical environment as you can get anywhere in the universe — and randomness is the same thing as entropy.