In an essay I wrote 24 years ago, I speculated about the possibility of life inside the sun. I’m sure that sounds crazy because everybody thinks in terms of life as we know it: water-based life relying on the chemistry of carbon. But things like water and chemistry are of secondary concern; if you truly understand the nature of life, you’ll realize that the true essence of life is negentropy. All living systems harvest negentropy to maintain or increase their orderliness.
Any living creature needs two things: a source of negentropy, and a mechanism for harvesting that negentropy. On earth, the most common source of negentropy is the sun, but there’s a second level of negentropy consisting of the plant material harvested by herbivores. A third level is the bodies of other creatures harvested by carnivores. There is also a source of negentropy provided by concentrations of some chemicals excreted from underground sources, such as hydrothermal vents.
The surface of the earth is an ideal location for the rise of life, because it enjoys a massive income of negentropy from the sun. This is crucial: it’s not the energy of sunlight that makes life possible, it’s the negentropy. What’s the difference between energy and negentropy? Think of it this way: imagine an earth surrounded by a dozen suns all around it. This earth would receive twelve times as much energy as our earth receives, yet it would not be able to host life, because it would be horrendously hot. Our earth sustains life because it has light and dark: during the day it receives sunlight, and at night it cools off. It is the huge difference between the intense sunlight of day and the blackness of night that constitutes the negentropy we receive. The huge difference between all this light and dark is an orderliness that makes life possible.
Scientists are hopeful that they’ll find life in the oceans of Europa, a moon of Jupiter. Why? Because it has water. Water is part of the mechanism for harvesting negentropy, but it’s not the driving force behind life. That driving force is negentropy. What sources of negentropy are there in the oceans of Europa? Certainly not sunlight — the water is under a thick layer of ice. There is some negentropy from possible hydrothermal vents on the sea floor, but it’s not much to work with. The hydrothermal vents on earth support ecosystems, but there’s a major question as to the genesis of these ecosystems. On the one hand, some scientists claim that hydrothermal vents provided an ideal environment for the genesis of all life on earth. This hypothesis is highly controversial. It is also possible that primitive life forms did indeed originate around hydrothermal vents, but then migrated to the surface, where they were able to harvest the immensely higher amounts of negentropy from the sun, kicking off the evolution of complex life forms, some of which returned to hydrothermal vents. The problem with these notions is that the transition from a metabolic system harvesting chemical negentropy to one harvesting sunlight is similar to the transition from a coal-burning locomotive to a Tesla. It’s just too big a leap to be plausible.
There’s another source of negentropy, though, that has not received due consideration: the interior of the sun. Here’s an image from NASA showing the interior of the sun:
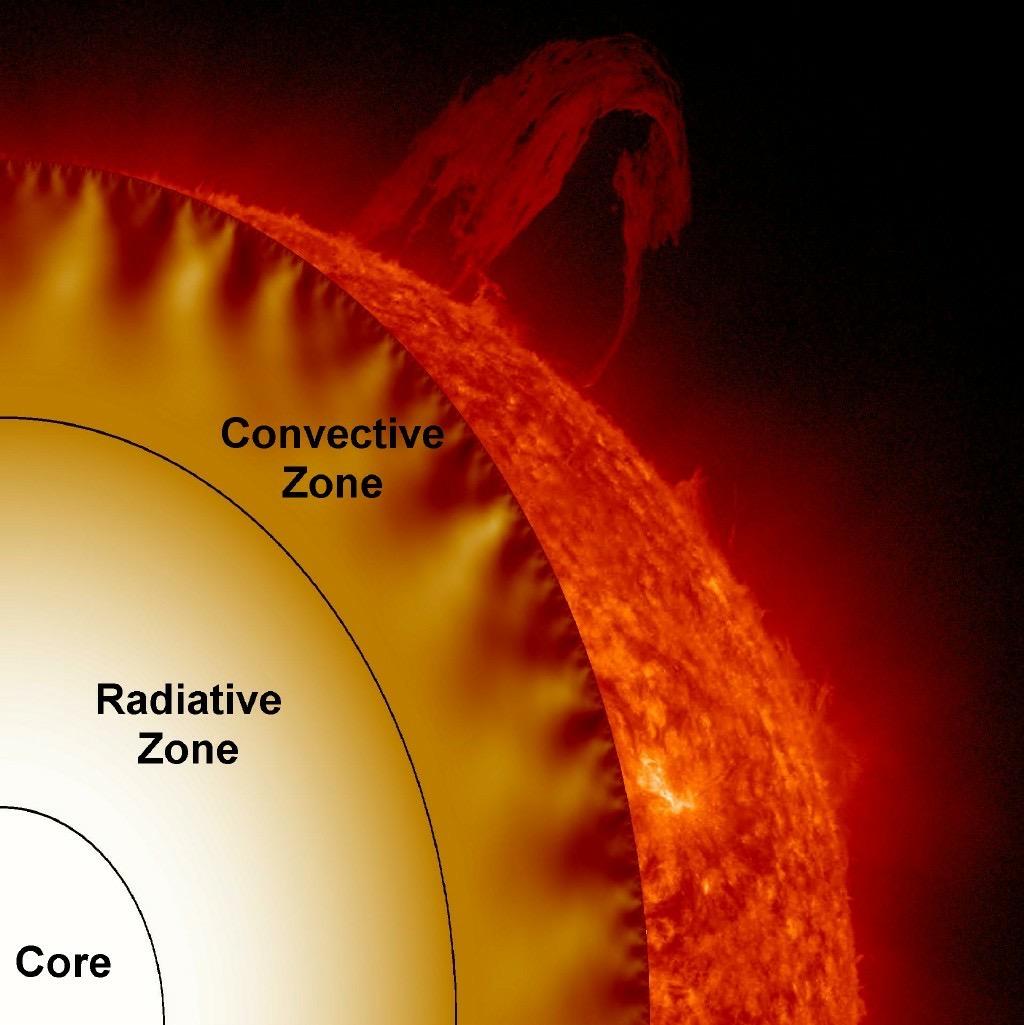
Energy is generated by thermonuclear reactions in the core, where hydrogen is transformed into helium, releasing stupendous amounts of energy. That energy takes the form of very high energy gamma photons. These photons radiate out from the core towards the surface. Along the way, they collide with protons, neutrons, and electrons, bouncing around from one to the next. Those protons, neutrons, and electrons are so densely packed that it takes about 100,000 years for a gamma photon traveling at the speed of light to reach the surface. (There are also some nuclei of larger atoms, but they are a tiny minority whose importance I’ll explain later.)
Now, there are two ways that energy is transferred from the core to the surface: radiation and convection. Radiative transfer is accomplished by photons traveling outward. By the time that these photons have burrowed their way to the upper edge of the radiative zone, they have lost so much energy from the zillions of collisions they have suffered that they are no longer the primary source of energy transfer. In the convective zone, energy is transferred primarily by, you guessed it, convection. The plasma inside the sun consists of protons, neutrons, and electrons bouncing around at extremely high temperatures — that is to say, moving very fast. The photons from the core keep kicking the particles around so powerfully that they get very, very hot. The fast-moving particles collide with other particles, transferring some of their energy to those other particles. To visualize this process, imagine a bunch of bumper cars colliding. If a really fast bumper car hits a slow-moving bumper car, the fast one loses some energy and goes slower, and the slow one gains some energy and goes faster. The same thing happens in the sun and is called ‘convection’.
Here’s the trick:
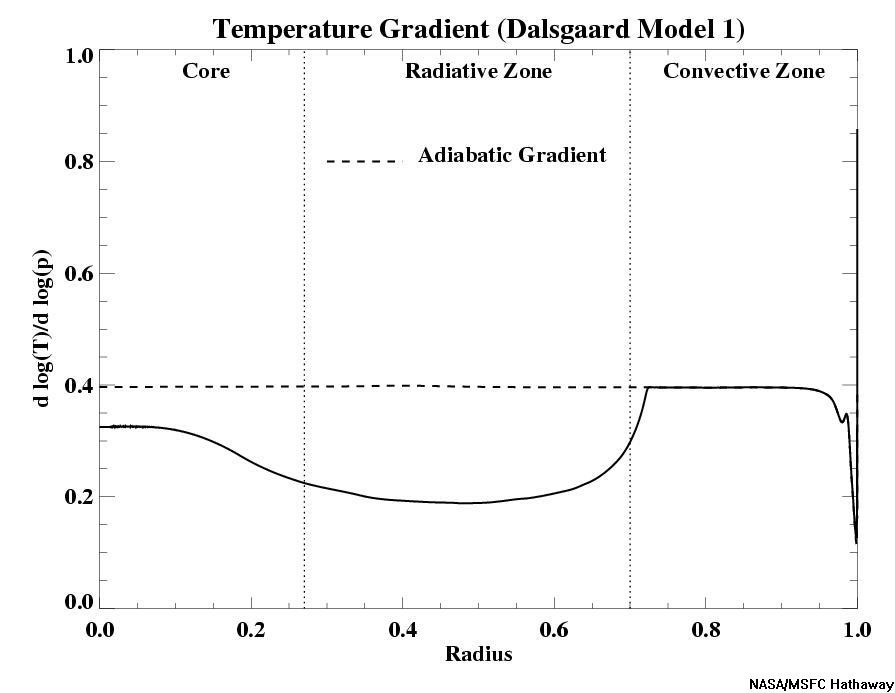
This shows the temperature gradient inside the sun. Where the temperature gradient is low (inside the radiative zone), the energy transfers efficiently. But there’s a kink in the graph at about 72% of the way from the center of the sun to the surface. That kink marks the point where the temperature of the plasma falls below about 2 million Kº. At that temperature, some of the larger atoms are able to retain some of their electrons. They have what’s called a large ‘cross section’ — from the point of view of the photons, they’re really big and so they present a major obstacle to the photons. At this point, the photons just aren’t very efficient at moving the energy outward; now the energy is carried outward by convection: the motion of the plasma itself. The hot plasma rises towards the surface of the sun; when it reaches the surface, it looks like this:
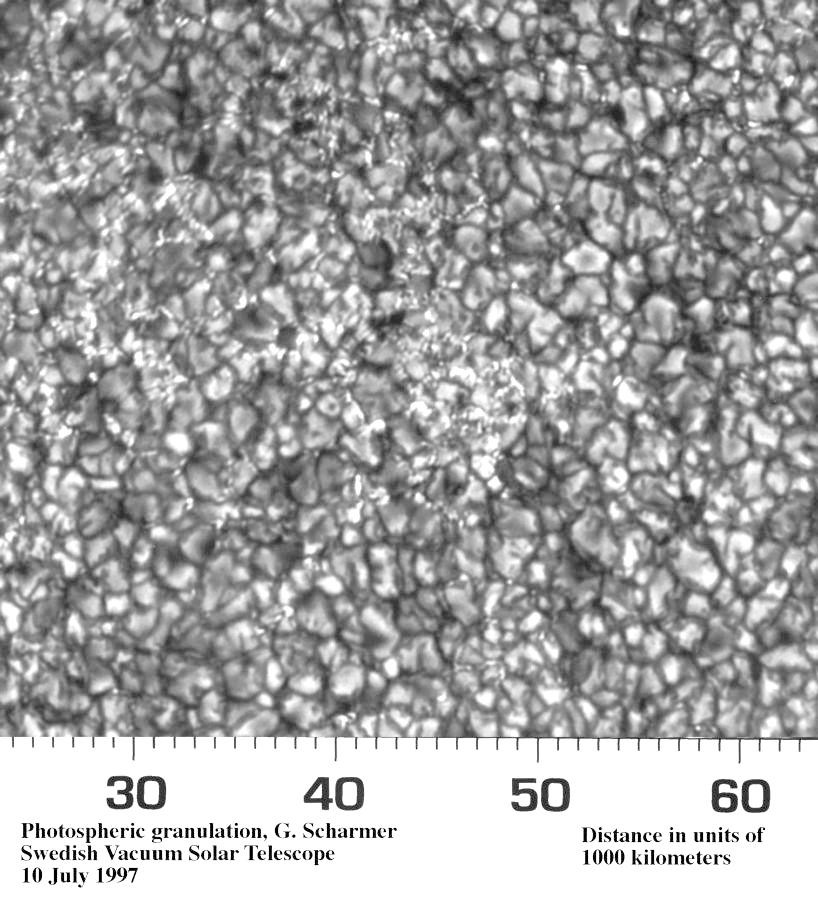
The little blobs are called ‘granules’ and are the tops of the rising convection cells. Here’s a movie showing how active this process is (the movie is speeded up). The hot stuff from below is rising; when it reaches the surface, it cools and moves downward along the dark spaces between the granules.
I direct your attention to the kink in the graph above; that’s where life might exist. That’s because negentropy is concentrated there. The outgoing photons are piling up in a traffic jam caused by the obstructing atoms that are not completely ionized, but convection is not yet developed fully enough to efficiently transfer the heat away. That transition zone from radiative transfer to convective transfer is characterized by strong differentiation: negentropy.
So we do have a source of negentropy. I’ll concede that the mechanism creating that differentiation is not as clearcut as what we have on earth. One way of thinking about negentropy is to think in terms of temperature differences. The sunlight hitting the earth is at an “internal temperature” of about 5000Kº, while the plants it hits are at an internal temperature of 300Kº. That temperature difference (5000Kº - 300Kº) represents the negentropy.
The temperature difference at the transition zone is not so stark, but it’s still big enough to be significant. More important is the fact that the radiative energy at that level is much, much greater than the sunlight that reaches the earth. If the sunlight were a million times brighter and the temperature difference were a million times smaller, you’d still get the same amount of negentropy. And the intensity of the radiation at that point inside the sun is very, very high.
OK, you give up — you’re willing to admit that there’s enough negentropy to drive living systems inside the sun. There’s still the second requirement: a mechanism for harvesting the negentropy. It certainly won’t be chemical; it’s too hot for any chemical reactions.
I propose magnetohydrodynamics instead of chemistry as the mechanism for harvesting negentropy. Magnetohydrodynamics is the study of the behavior of electrically conductive fluids, such as plasmas. Check out the link to Wikipedia. Ack! No, I don’t get it, either. I understand magnetohydrodynamics at a qualitative level; the mathematics goes way over my head.
Here’s how to think about it: imagine a collection of free-moving charged particles: protons and electrons. Because they are much lighter than the protons, electrons move much faster than protons. So these are a bunch of billiard balls bouncing around against each other. Pretty simple, huh?
The Catch
The catch here is that a moving charged particle generates a magnetic field — and magnetic fields influence the motion of charged particles. In other words, every single moving electron and every single moving proton generates a magnetic field that alters the motion of every other moving electron and proton. Everything that’s happening is changing everything else that’s happening! Arg!
At the macroscopic level (big) you can use statistical methods to figure out a lot of the behavior of a plasma, but the killer problem is at the microscopic level. With small groups of charged particles, fluctuations are inevitable, and a particularly strong fluctuation can push things off in an unexpected direction, triggering a much larger fluctuation. This is the problem that has kept tokamaks from working for over 70 years now. In the sun, though, I suspect that a statistically highly unlikely fluctuation could lead to a self-perpetuating cyclic process that could provide the seed for a growing collection of such self-perpetuating processes that could eventually converge into something quite large and complicated.
Yes, such a development is highly unlikely. But remember that the rise of life on earth was also highly unlikely, and that the sun has 8,000 times as much surface area as the earth to experiment with.