Let’s start with the first animals swimming around in shallow Ediacaran seas some 600 million years ago. We don’t know much about them, because they were all soft-tissue creatures, which left very little in the way of fossil remains. This artist’s rendering is based on what we do know:
Note that the animals in this picture come in two basic forms: jellyfish and worms. (Not at all like modern jellyfish or worms, but that’s the closest thing we have to these weird creatures.) Now, the big thing that these animals had was mobility: they could move around by using a newfangled invention, the muscle cell. However, a single muscle cell wasn’t powerful enough to propel one of these critters, so they needed entire clusters of muscle cells working in tandem like rowers on a scull. The “worms” managed this by the simple expedient of lining up their muscle cells like so:
This arrangement is, as Spock would say, “crude but effective”. When a muscle on the left end contracts, the contraction eventually tugs on the next muscle in the line, which triggers it to contract, which later tugs on and triggers the next muscle, and so on. The contraction travels down the line of muscle cells rather slowly because the muscle cells themselves are rather slow to operate. In the process, the contraction tends to curl or curve the flesh of the worm, like so:
It’s just like the way your skin puckers when you pinch it: the contraction forces it sideways. Thus, the worm writhes in a slow wavelike motion that propels him through the water. This motion doesn’t require much in the way of control circuitry: each muscle cell serves as the controller for the next cell, so the system is in effect self-regulating.
The jellyfish expanded this basic system into a two-dimensional circular arrangement:
All this needed was a central timing unit that triggered the top end of each chain of muscle cells; the wave of contraction would propagate down all the chains, causing the jellyfish to undulate in a properly jellyfishlike manner.
The Cambrian Explosion
About 543 million years ago, something amazing happened. We still don’t quite know exactly what it was, but it seems that animals suddenly stumbled upon the magic ingredient for success, and as a consequence, there were suddenly a zillion strange new creatures swimming around in those shallow seas, and they apparently gobbled up most of the old-style jellyfish and worms.
What was this magic ingredient that set life on fire (not literally) and spawned such a crazy parade of new creatures? The best explanation offered so far comes from Andrew Parker, who collected the evidence to demonstrate that it was the development of vision that triggered the Cambrian Explosion. Before then, animals were blind, and it’s really hard to do anything useful with mobility if you can’t see where you’re going. But there was another huge difference between Ediacaran life and Cambrian life, a difference more fundamental than vision: nervous systems, that is to say, groups of neurons that establish communications between various parts of the body. With the Ediacaran life, it was literally true that the left hand (or glob of tissue) didn’t know what the right hand was doing. It wasn’t necessary: Ediacaran fauna got along just fine without the different parts of their bodies communicating with each other. But Cambrian fauna had something very different: differentially moving parts. Consider, for example, the trilobite in the picture above (he’s the guy in the bottom center who looks vaguely like a beetle). All those busy little legs can’t just wiggle around randomly; for the trilobite to make any forward headway, the legs must be coordinated in their movement. Some master control center (a brain) has to send out signals to all the legs, telling which ones to move forward and which ones to move backward. And those signals have to be carried from that brain to those muscle cells. What carries those signals? The neuron, which I believe to be the first and fundamental invention that triggered the Cambrian Explosion. The basic design of a neuron is quite simple: it’s nothing more than a biochemical wire that transmits electrochemical signals from one end to the other end:
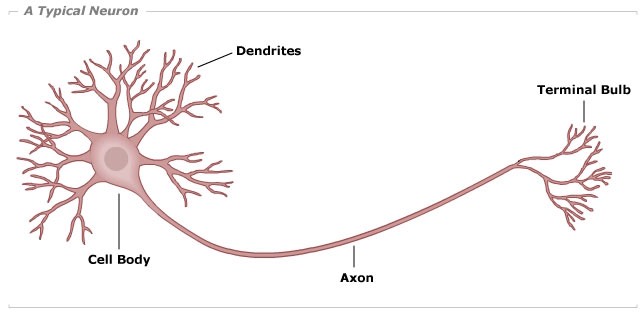
(Credit: Pennsylvania State University)
I suspect that the neuron evolved from the muscle cell, because the electrochemical signal that one muscle cell transmitted to the next muscle cell was the key trick that the neuron needed. So you start with a muscle cell, delete the ability to contract but retain the ability to have an electrochemical signal traverse the length of the cell, and you get a neuron.
Although most trilobites had eyes, some orders of trilobites were eyeless. It certainly seems reasonable to suggest that some trilobites could get along just fine without vision, using their little feelers to figure out where to go. Eyes were certainly useful -- but nervous systems had to precede eyes.
But exactly how were eyes and nervous systems integrated? After all, the eyes might be able to detect varying amounts of light, but unless the creature can interpret those images in terms that are useful, eyes don’t do much good. So, how were early optical systems organized? Of course, we have no empirical data on this -- no trilobite nervous systems have survived the last half billion years. However, based on what we know about the vastly more complex visual systems of modern animals, we can come up with a reasonable surmise as to the basic principles at work.
Let us use as our hypothetical example, little Tommy Trilobite. He has a simple visual system consisting of a low-resolution compound eye (such as insects have nowadays). For even greater simplicity, we’ll posit that Tommy has only four photoreceptacles in his eye; in other words, his visual field looks something like this:
Suppose further that Tommy sees in black and white with no grey. In other words, he perceives each circle as either white or black. (I’m really simplifying matters here!) Lastly, suppose that Tommy has just three behavioral responses to his visual inputs: 1) he does nothing because there’s nothing happening in his visual field; 2) he sees a small creature moving so he moves to gobble it up; 3) he sees a big creature moving, which could well be a predator such as the Anomalocarus shown swimming over the trilobite in the illustration above, so he runs for his life. Here’s a schematic for the neurons that process this data:
The magenta circles represent the signals coming from the four visual receptors in the eye; they’re either off or on. The red neuron triggers the neurons that start the processing of running away; the blue neuron triggers the process to chase after something to eat it. Each arrow represents an “excitatory input” -- a signal that will cause the receiver neuron to trigger, but ONLY if one or more other signals are received at the same time. In other words, a receiver neuron will only trigger if it receives at least two votes from two different neurons. The really big arrowhead counts as four votes.
The green neuron (“something moved!”) takes a little more explanation. It is actually ten different neurons, one for each ordered pairing of magenta neurons. Here’s what just one of those neural circuits looks like:
Again, the magenta neurons A and B represent any pair of neurons coming from the eye. We have one set of these for each of the following pairs of neurons: A and B; B and A; A and C; C and A; A and D; D and A; B and C; C and B; C and D; D and A. The trick here is in the purple “slow neuron”. If something appears in cell A, it sends a signal to the slow neuron, which takes its sweet time before passing the signal on to the big green neuron. If in the meantime something has also appeared in cell B, then a signal goes directly from cell B to the big green neuron. If the timing is right, then both signals will reach the big green neuron at the same time, which will be sufficient to trigger it.
This neural circuit, then, will trigger the red neuron (“run for your life”) if something appears in all four cells -- and something that big can only mean trouble. But if something appears in one cell and then moves to another cell, then it’s something small and moving: food. This system, then , can distinguish between two very simple visual patterns. The basic design is extensible: more complex patterns can be recognized by simply adding more neurons in more complicated connection arrangements. You could get a neural circuit that can tell the difference between these two visual patterns:
Extend it they did, as animals built ever more complex brains capable of recognizing all sorts of subtle visual patterns. They even integrated multiple sensory inputs to further refine the pattern recognition, as in the old joke “looks like moose shit... feels like moose shit... smells like moose shit... tastes like moose shit… ya think it’s moose shit?”. They also speeded up neurons for faster processing and made them capable of connecting to and receiving from hundreds of other neurons. And so animals just got better and better, and the Darwin-Jones index reached record highs. This takes us up to about the early Triassic roughly 200 million years ago.
Our next exciting episode: Sequential Thinking Appears!